When the Blue Origin space flight blasted off on Dec. 19, it carried three UCF-led research studies aimed at keeping astronauts and equipment safe, and understanding the formation of our solar system.
This is the sixth time UCF has had experiments fly aboard New Shepard, with previous flights in August 2021, January, May and December of 2019 and April 2016. The studies are among several dozen research payloads on NS-24, New Shepard’s 24th mission.
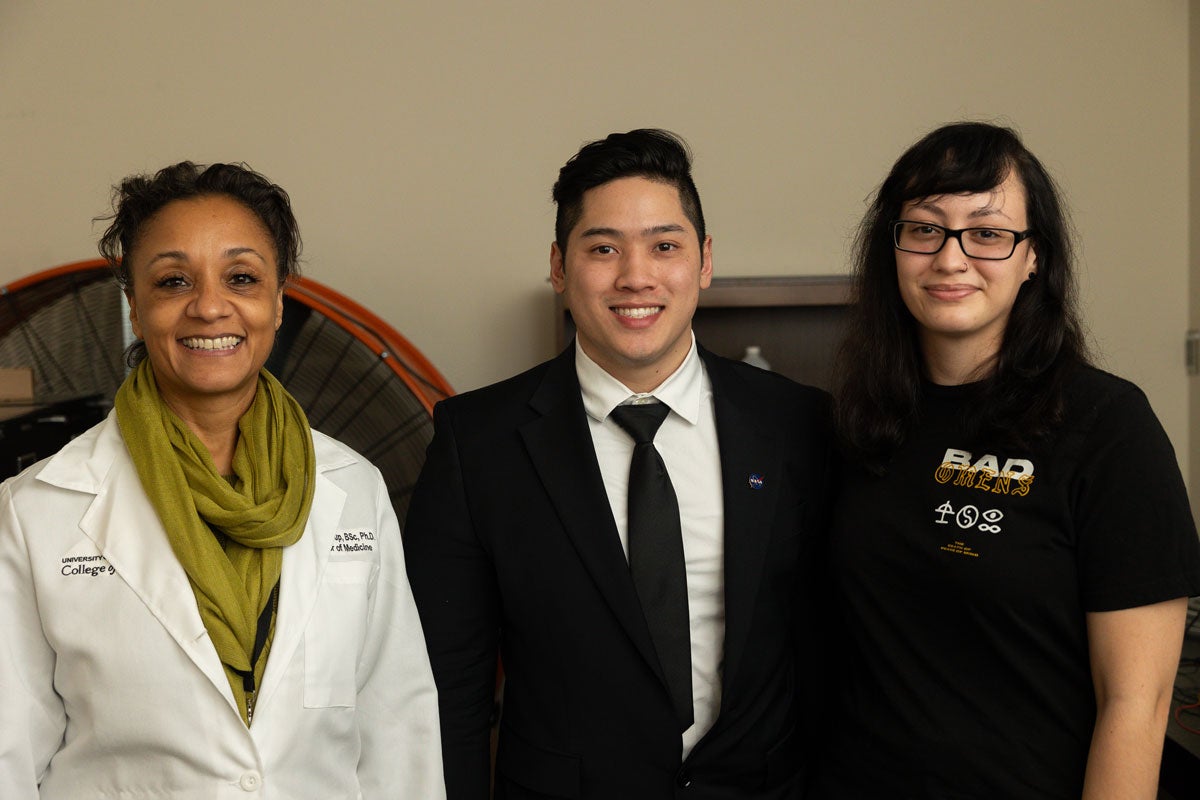
Bone Loss in Space
UCF College of Medicine biomedical engineer Melanie Coathup is partnering with Michael Kinzel, an associate professor in UCF’s Department of Mechanical and Aerospace Engineering, to understand how the absence of gravity in space impacts the bones of space travelers.
“When you’re in microgravity, there’s a change in characteristics of fluid flow and we’re trying to find out if that includes the fluid flow in our bodies, particularly through our bones,” says Coathup, who heads UCF’s Biionix faculty cluster initiative, an interdisciplinary team developing innovative materials, processes, and interfaces to support health and well-being.
Microgravity-induced bone loss is a health risk for space travelers and long-term goals of human space exploration and colonization. NASA research has shown that astronauts who stay in space for extended periods can lose up to 1% to 2% of bone density per month, primarily in weight-bearing bones like the spine, hips and legs. That compares to bone loss of 0.5% to 1% per year in aging men and post-menopausal women on Earth. This significant bone loss can place space travelers at risk for bone fracture and an early-onset spaceflight-induced osteoporosis.
Coathup theorizes that while on Earth, gravity exerts a constant mechanical load to the skeletal system whenever we sit, walk or stand, which causes a tiny amount of fluid to flow in and out of bones.
“On Earth, when we bear weight on our bones, it forces fluid into the tissue and then as we take off the ground, water draws back out. So that applies a mechanical stimulus to our cells that sends nutrients into the bone and then removes waste products as well,” Coathup says.
“We predict that in microgravity, in the absence of weight-bearing, there’s very little fluid movement, which stops or reduces that mechanical stimulus that sends nutrients in and stops waste products from going out and we believe this may contribute to bone damage,” she says.
This New Shepard mission does not have crew, but human subjects are highly complex and difficult to understand. So, the UCF researchers are combining medical and mechanical engineering technology to develop novel models that directly focus the study of human bone behavior to fluidic character in microgravity. Coathup is gathering the small-scale porous structure of bones using medical technology (CT Scans). These geometries are being used by Kinzel’s group to create a microfluidic chip to represent this geometry. These microfluidic chips are miniature flow channels that include artificial capillaries using advanced 3D printing technology. Fluid and tiny beads are pumped through the chip to mimic the proteins and solutes in blood. The goal is to develop comparisons of the flow in these bone-like chips in microgravity to the behavior in various orientations in normal gravity on Earth.
“We expect to see that the presence of gravity enhances micro-scale mixing needed to support healthy bones,” Kinzel says.
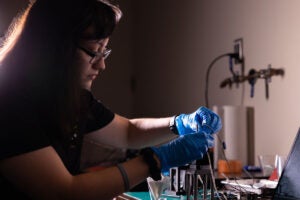
The experiment is not a perfect representation of a real bone, but rather a simplified model to help researchers understand mechanisms. The size of the beads and capillaries are much larger in the experiment than in people’s bodies.
“To accommodate this, we plan to use high-end computational modeling to scale down the experiment to a real human bone,” Kinzel says.
Kinzel, an expert in computational fluid dynamics, leads the team that is creating the microchip and its platform.
The overall study is led by imec, an international nano- and digital technology research organization. The study is primarily focused on demonstrating imec’s lens-free microscope technology in a space environment. UCF and imec collaborated to formulate research questions to demonstrate the added value of these new microscopes, which are both smaller and lighter than conventional ones, for future biological testing on the International Space Station.
Coathup has dedicated much of her research to figuring out how bones are impacted by aging and environmental stressors such as space flight, and is working on developing new technologies and therapies that can protect, repair or rebuild damaged bones.
Her collaboration with Kinzel is one of numerous payloads on this flight funded by NASA primarily through the NASA Flight Opportunities program. These payloads are helping researchers better understand the capabilities of living and working in space.
Dust Behavior in Microgravity
This project — titled Electrostatic Regolith Interaction Experiment (ERIE) — examines charged dust behavior in microgravity and also tests sensors that will characterize the charging behavior of dust in a lunar-like environment.
Key to these experiments is the several minutes of microgravity provided by the Blue Origin flight.
The research is led by Adrienne Dove, an associate professor in UCF’s Department of Physics, in collaboration with researchers at NASA’s Kennedy Space Center.
The sensors are being developed by collaborators at Kennedy to be used on lunar missions, such as on rover wheels, where they could measure charge on dust grains in natural lunar environments.
The results can inform strategies to keep lunar dust from damaging electronics, solar cells and mechanical equipment, and even human suits and systems during lunar missions.
The research is funded through NASA’s Flight Opportunities Program within its Space Technology Mission Directorate.
Seismic Wave Propagation in Asteroids
This project, titled Microgravity Experiment for the Speed of Sound (MESS), is examining how seismic waves and shaking can modify the surface and interior of an asteroid, and impact-induced seismicity can dictate surface features as well as overall shape and compactness changes within the asteroid.
The research is led by Julie Brisset, a research scientist and interim director of the Florida Space Institute (FSI) at UCF. Brisset researches dust behavior under microgravity conditions for the study of planet formation and regolith.
This work is important since the mechanical structure and dynamical behavior of small asteroids can retain clues to the early processes taking place during planet formation times.
In this experiment, simulated asteroid granular material that had been placed into three separate containers has sound waves generated into them, and their speeds are recorded while in the microgravity environment of the New Shepard rocket.
Three types of asteroid material simulant are used in the experiment: fine grains (about 0.1 millimeter), millimeter-, and centimeter-sized grains.
These simulants are routinely prepared at principal investigator Brisset’s lab at UCF’s Florida Space Institute.
Undergraduate students assembled the payload, and over the course of its design and implementation, a total of about 10 undergraduate students participated for a project duration of five semesters, with graduating students training new arrivals on the team.
“Students were not only trained in hands-on skills in their respective areas of expertise and integrated teamwork, but also in mentoring and project management as well,” Brisset says. “They learned to handle deadlines and project documentation, and overall, had an exceptional experience preparing them for their post-graduation professional life.”
Researchers’ Backgrounds
Coathup received her doctorate in orthopedic implant fixation from University College London and joined UCF in 2017. Before coming to UCF, she worked at the University College of London for 17 years. Her work includes the development of a novel synthetic bone substitute material Inductigraft to boost bone repair and regeneration, which is mainly used in spinal fusion surgery and marketed by Baxter Healthcare. Her research excellence has been recognized internationally through her publications and through receiving several prestigious UK, European and international prizes from her peers.
Kinzel received his doctorate in aerospace engineering from Pennsylvania State University and joined UCF in 2018. In addition to being a member of UCF’s Department of Mechanical and Aerospace Engineering and a part of UCF’s College of Engineering and Computer Science, he also works with UCF’s Center for Advanced Turbomachinery and Energy Research.
Dove received her doctorate in astrophysical and planetary sciences from the University of Colorado at Boulder and her bachelor’s degree in physics and astronomy from the University of Missouri. She joined UCF’s Department of Physics, part of the College of Sciences, in 2012. In 2017 Dove was awarded the Susan Niebur Early Career Award by the NASA Solar System Exploration Virtual Research Institute (SSERVI) for her contributions to the science and exploration communities. She has also received UCF’s Reach for the Stars Award and Luminary Award.
Brisset earned her master’s degrees in aerospace engineering in 2005 from the Institut Supérieur de l’Aéronautique et de l’Espace in Toulouse, France, and the Technical University of Munich. After working for several years as an aerospace engineer on European Space Agency International Space Station payload operations, she started graduate studies in astrophysics at the University of Braunschweig, Germany and received her doctoral degree in 2014.